Fusion’s New Hero: The Hottest Liquid Metal
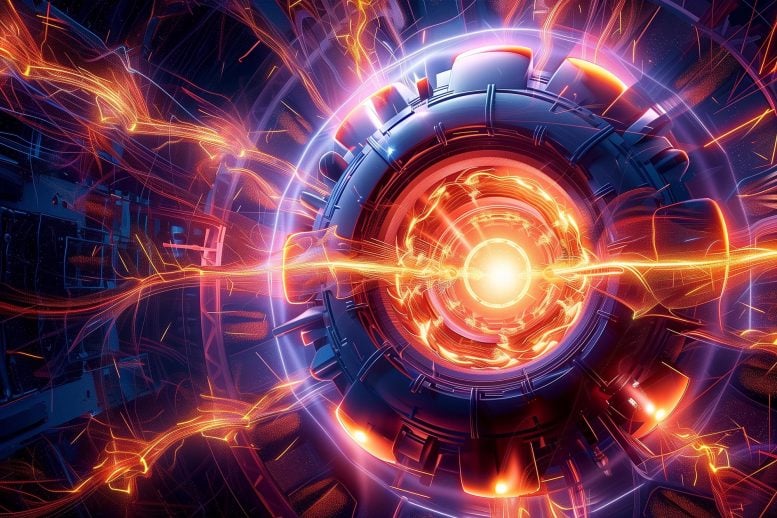
Scientists at the Princeton Plasma Physics Laboratory are beginning to use liquid lithium in spherical tokamaks to improve the fusion process.
Recent computer simulations suggest the precise placement of lithium vapor to protect the tokamak’s interior from energization. plasma temperature. New configurations, such as the “cave” of lithium and walls with porous plasma, aim to simplify the design and improve heat dissipation, contributing to the future of fusion energy.
Inside the next generation of fusion vessels known as spherical tokamaks, scientists at the US Department of Energy’s Princeton Plasma Physics Laboratory (PPPL) envisioned a hot spot containing a metal flowing that reminds us of an underground cave. The researchers say that the vaporized liquid metal can protect the tokamak’s interior from the intense heat of the plasma. It’s an idea that dates back several decades and is linked to one of the Lab’s strengths: working with wet metals.
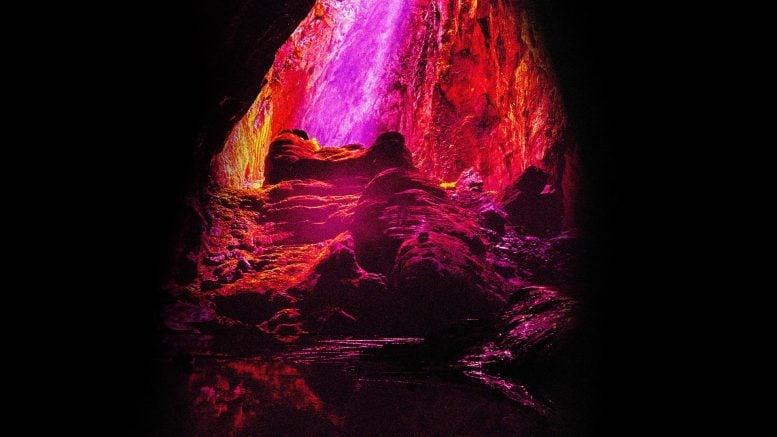
Advanced Liquid Tokamak Design
“PPPL“The knowledge of using liquid metal, especially liquid lithium, for improved fusion processes is helping to improve ideas on how it can be used in a tokamak,” said Rajesh Maingi, head of PPPL of experimental tokamak science and co-author of a new paper Nuclear Fusion which describes the construction of the lithium deposit.
Recently, researchers have been doing computer simulations to find the perfect location for the lithium vapor “cave” inside the fusion vessel. To achieve commercial fusion, each part of the donut-shaped tokamak needs to be properly positioned. The idea behind the lithium vapor cave is to store lithium at the boundary layer away from the hot, condensing plasma but close to extreme temperatures. The evaporator – a heated place for boiling lithium atoms – places the lithium vapor particles in the right direction where most of the excess heat tends to accumulate.
Scientists considered three options for placing the cave. A lithium vapor cave could exist below the tokamak near the central core, in an area known as the independent flux zone; it can be on the outer edge, known as the normal flux area; or lithium vapor can come from both places.
Now, the results from many computer simulations have determined that the best place for the lithium vapor cave near the bottom of the tokamak is the center. The new simulations reveal more information: They are the first to consider collisions between neutral particles, which have no positive or negative charge.
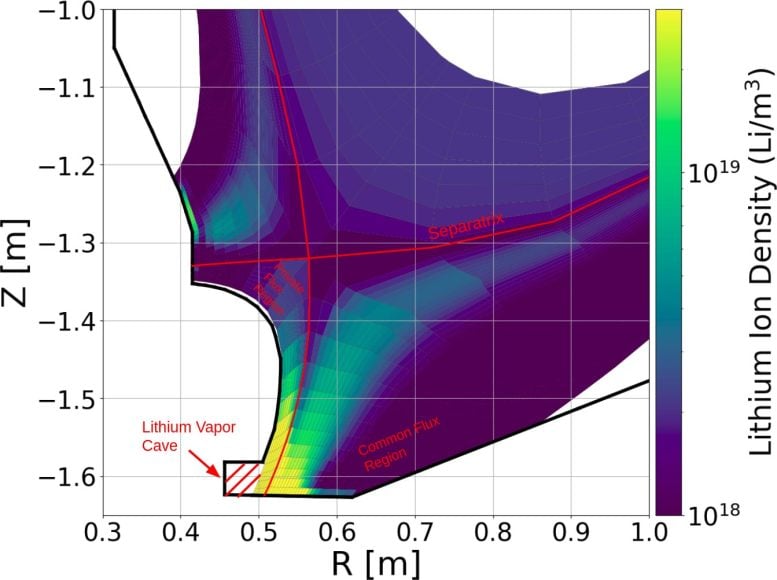
Advantages of Putting Lithium in Private Flux
“The lithium evaporator doesn’t work unless it’s placed in a private space,” said Eric Emdee, a research physicist at PPPL and lead author of the new paper. When lithium vaporizes in a private space, the particles become positively charged ions in a superheated space, protecting the surrounding walls. Once the lithium particles are ionized, they obey the same gravity as the plasma, they spread and destroy the heat thus hitting a larger area of the tokamak and reducing the risk of the particles melting.
The private flux region is also an ideal target for evaporated lithium because it is separated from the core plasma, which needs to stay hot. “You don’t want your core plasma to be contaminated with lithium and cool, but you also want the lithium to cool down before it leaves the cave,” he said.
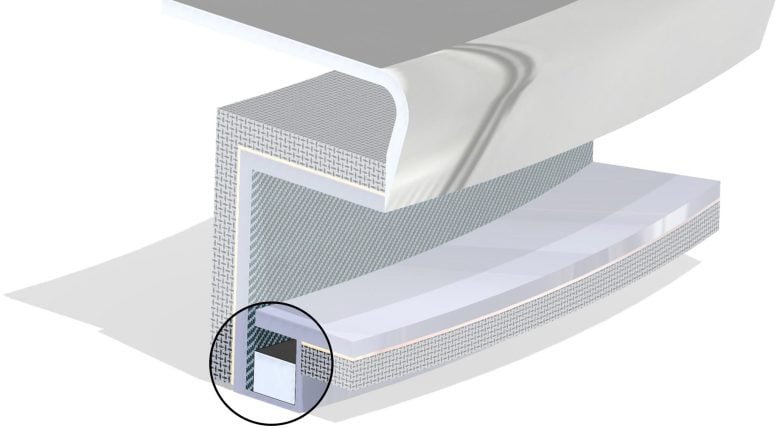
Analyzing Lithium Containment: From Box to Cave
The researchers initially thought that lithium could be best placed in a “metal box” with an open top. The plasma would flow through the gap so that the lithium could dissipate the heat of the plasma before it reached the metal walls. Now, the researchers say that the cave – geometrically only the inner half of the box – filled with lithium vapor can be easier than the box. The difference isn’t just semantics: It affects where the lithium goes and how efficiently it dissipates heat.
“For many years, we thought we needed a box full of four sides, but now we know we can make something simpler,” said Emdee. The data from the new simulations pointed them in a different direction as the research team realized that they could have more lithium if they cut their box in half. “Now we call it the cave,” said Emdee.
In the cave configuration, the device would have walls at the top, bottom and side near the center of the tokamak. This improves the way the lithium evaporates, putting it in a better way to capture more heat from the private sector while reducing the complexity of the device.
New Methods of Temperature Control
However, another method proposed by PPPL scientists in a new paper can achieve the same effect of extinguishing heat without significantly changing the shape of the tokamak wall. In this method, liquid lithium flows rapidly under a porous wall, facing the plasma. This wall would be where the extreme heat affects the tokamak the most: the divertor. The porous wall allows lithium to enter the surface of the water directly facing the temperature of the plasma, so the liquid lithium is supplied exactly where it is most needed: in the area of the highest temperature. This porous capillary system was described in an earlier paper published in the journal Physics of Plasmas.
The lead author of the paper, PPPL Principal Engineering Analyst Andrei Khodak, said he prefers the idea of using a wall that faces the plasma itself, as the tiles are inserted into the tokamak. “The advantage of the porous wall facing the plasma is that you don’t need to change the shape of the vessel being closed. You can just change the tile,” said Khodak. Khodak was also a co-author on the new paper, along with former Lab Director Robert Goldston.
Having the evaporation of lithium on the divertor leads to a strong coupling between the plasma boundary and the part facing the plasma in terms of heat and mass transfer because the heating from the plasma will lead to the evaporation of lithium, which which will change the temperature of the plasma. in the liquid phase facing the lithium plasma. The new model, described in a paper by the same authors in IEEE Transactions on Plasma Science, accounts for the dynamic coupling of the two methods. PPPL’s scientists and engineers will continue to test and improve their ideas as part of their ultimate goal of making composites an integral part of the power grid.
Reference: “Optimization of lithium vapor box divertor evaporator location on NSTX-U using SOLPS-ITER” by ED Emdee, RJ Goldston, A. Khodak and R. Maingi, 2 July 2024, Nuclear Fusion.
DOI: 10.1088/1741-4326/ad57d2
DOE supported the work on the new Nuclear Fusion paper under contract number DE-AC02-09CH11466. The Physics of Plasmas manuscript was based on work supported by the DOE, Office of Science, Office of Fusion Energy Sciences and was written by Princeton University under contract number DE-AC02-09CH11466.
#Fusions #Hero #Hottest #Liquid #Metal